| |
| Med Sci (Paris). 34: 81–86. doi: 10.1051/medsci/201834f114.Overexpression of RBM10 induces osteosarcoma cell apoptosis and inhibits cell proliferation and migration Li-ping Han,1 Cun-ping Wang,1 and Si-lin Han1* 1Department of Orthopaedics, Zaozhuang Municipal Hospital, 41 Longtou Rd, Zaozhuang277100, Shandong, P.R. China |
Osteosarcoma is the most common malignant bone tumor with high incidence in adolescence and poor prognosis. RBM10, a member of RBPs, was reported to be a tumor suppressor in many kinds of cancers. However, the roles of RBM10 in osteosarcoma remain unknown. In this study, we found that overexpression of RBM10 decreased osteosarcoma cell proliferation and colony formation in soft agar, and inhibited osteosarcoma cell migration and invasion. Our results also revealed that RBM10 overexpression induced osteosarcoma cell apoptosis via the inhibition of Bcl-2, the activation of caspase-3, and the transcription and production of TNF-α. Our results indicated that RBM10 acts as a tumor suppressor in osteosarcoma. This could enable to define a new strategy for diagnosis and treatment of patients with osteosarcoma. Keywords: osteosarcoma, RBM10, tumor suppressor, cell proliferation, cell apoptosis |
Osteosarcoma is the most common malignant bone tumor, characterized by high incidence in adolescence, early lung metastasis and poor prognosis [1]. In the past decades, great progress has been made in the development of radical surgery and neoadjuvant chemotherapy [2- 4]. However, more than 20% of osteosarcoma patients die from pulmonary metastases or tumor recurrence [2, 5]. Thus, it is urgent to explore novel strategies for early diagnosis, prognostic evaluation and bio-therapeutics in osteosarcoma to improve the current situation of diagnosis and treatment. RNA binding proteins (RBPs) are a large class of proteins involved in regulating the quantity and functionality of gene products [6]. RBM10, a member of RBPs, was first identified in 1995 [7]. It regulates the alternative splicing [8- 11] of NUMB [12], Dlg4 [10], FAS [11], Dnmt3b [13], and SMN2 [14] and is expressed in most mammalian cells [15]. However, RBM10 is mutated in a number of cells, which is associated with many diseases, including neurological, muscular, sensory or cancers [15- 22]. In recent years, it was reported that RBM10 was a tumor suppressor in many types of cancers, such as lung cancer and breast cancer [23]. Of note, overexpression of RBM10 increases apoptosis via elevated level of TNF-α mRNA and soluble TNF-α protein [24]. LUAD-associated RBM10 mutations, which exhibit a mutational spectrum similar to that of tumor suppressor genes, have been associated to a decrease in cell proliferation [25]. RBM10 also decreases colony formation and xenograft tumor growth in lung cancer [8, 12]. However, the role of RBM10 in cancer progression is controversial. Loiselle et al. reported that RBM10 expression contributes to tumor growth and metastasis in RBM5-null tumors [26], suggesting that it can also act as a tumor promoter. Surprisingly, there is no studies on the roles and mechanisms of RBM10 in osteosarcoma. In this study, we report that overexpression of RBM10 significantly decreases osteosarcoma cell growth, including cell proliferation and colony formation in soft agar, and inhibits osteosarcoma cell migration and invasion. Our results also revealed that RBM10 overexpression induces osteosarcoma cell apoptosis via the inhibition of Bcl-2, the activation of caspase-3, and the production of TNF-α. These results suggest that RBM10 acts as a tumor suppressor in osteosarcoma. and it might be a novel biomarker for diagnosis and represent a new avenue for the treatment of patients with osteosarcoma. |
Cell line, cell culture and cell transfection The osteosarcoma cell line U2OS was purchased from the CAS cell bank (Shanghai, China), and cultured in RPMI-1640 (HyClone) supplemented with 10% fetal bovine serum (FBS, Gibco) and 1% Penicillin-Streptomycin Solution (Sigma) (complete medium). For further experiments, U2OS cells were transfected with pcDNA3.1-RBM10 (Genechem, Shanghai, China) using lipofectamine 2000 according to manufacturer’s instruction. RNA extraction and real-time quantitative polymerase chain reaction RNA was isolated with a RNA extraction kit, and reverse transcription was performed to generate complementary DNA according to the manufacturer’s protocol (Cwbiotech, Beijing, China). RBM10 and TNF-α mRNA expression was measured by real-time quantitative polymerase chain reaction. β-actin was included as an internal reference. The relative expression was calculated using the 2 (-ΔΔCt) method. The primers were as follows: rbm10 forward: 5’- GGGGTGTCCTCTAACATTGG-3’, rbm10 reverse:5’-ATGGTCTTGCCGTCGATAGT-3’; tnf-α forward: 5’-GGAGAAGGGTGACCGACTCA-3’, tnf-α reverse: 5’-TGCCCAGACTCGGCAAAG-3’; β-actin forward: 5’-CCCGAGCCGTGTTTCCT-3’, β-actin reverse: 5’-GTCCCAGTTGGTGACGATGC-3’. Western blot Cells were lysed in the RIPA lysis buffer containing protease inhibitors (Cwbiotech, Beijing, China). The protein concentrations were measured using the Bicinchoninic Acid kit (Cwbiotech, Beijing, China). Protein samples (20 µg per lane) were subjected to SDS-PAGE and then transferred to a PVDF membrane. The membrane was blocked for 1 h at room temperature in 5.0% non-fat milk and then incubated with monoclonal antibodies against RBM10 (Abcam, 1:1000), Bcl-2 (PTG, 1:1000), caspase-3 (PTG, 1:1000) and GAPDH (PTG, 1:5000) at 4°C overnight. The species-relevant horseradish peroxidase-conjugated secondary antibody (PTG, 1:5000) was added after washing 3 times with TBS containing 0.1%Tween-20. The blots were visualized using an ECL assay. Cell proliferation assay CCK-8 assay was used to assess cell proliferation ability. In brief, cells were seeded in 96-well plates at a density of 1×10 3 cells per well and incubated at 37°C. At separate time points (1, 2, 3, 4, and 5 days), CCK-8 solution (10 μL, Solarbio, Beijing, China) was added into each well, and the cells were incubated for an additional 1.5 h at 37°C. Then, the absorbance of each well was measured at 450 nm using a microplate reader. All experiments were performed in triplicate. Cell invasion and migration assay 100 μL Matrigel (BD) were deposited into the upper chamber of Transwell (Millipore) and incubated in a 5% humidified CO 2 atmosphere for 2 h to be hydrated. 500 μL serum-free medium were then added into the bottom well and incubated for 30 min. Next, the medium in the upper chamber was removed and replaced with 100 μL cell suspension (1×10 5 cells) in complete medium. 500 μL complete medium were added in bottom well. After 24 h, cells on the upper surface of the filter membrane were swabbed with cotton swabs, and those on the lower surface of the filter were fixed with polyoxymethylene and stained with Giemsa for 5 min. Nine random fields per filter from three independent wells were photographed and counted. The cell migration assay was the same as the cell invasion assay except that the upper chambers were not coated with Matrigel. Colony formation assay RBM10-overexpressing U2OS cells (termed herein RBM10) and negative control U2OS cells (NC) were seeded in a 35 mm dish at a density of 600 cells/well in suspensions of 0.35% agar containing medium supplemented with 10% fetal bovine serum on top of a bed of 0.7% agar containing the same medium. Mediums were replaced every three days. After incubation for 14 days, colonies were fixed with 4% formaldehyde and stained with 0.5 mg/mL crystal violet. Images were taken, and the colonies were counted. The average numbers of colonies for both NC and RBM10 groups were calculated. All experiments were performed in triplicate. Cell apoptosis assay Cells were harvested, washed with pre-cooled PBS, and resuspended in binding buffer. Next, 5 μL of FITC-labelled Annexin V (4A Biotech, Beijing, China) were added to 100 μL cell suspension containing 1-5×10 6/mL and incubated at room temperature in the dark for 5 min. After incubation, 10 μL of Propidium Iodure (PI) were added, and the apoptotic and necrotic cells were quantified by flow cytometry. ELISA The production of TNF-α was detected using a TNF-α ELISA (PTG, KE00068) kit according to manufacturer’s instruction. Statistical analysis All data were analyzed by using the SPSS18.0 software. The experimental data are represented as mean ± SD. Comparison of different groups were determined by Student’s unpaired t-test. P<0.05 was considered to be statistically significant. |
Overexpression of RBM10 inhibits the proliferation of U2OS osteosarcoma cells. Cancer progression is a complex process involving cell growth, migration, invasion, colony formation, and metastasis [ 27], [ 28]. To investigate the role of RBM10 in osteosarcoma cell proliferation, we first transfected osteosarcoma cell line U2OS with pcDNA3.1-RBM10 to overexpress RBM10. The overexpressed efficiency was assessed at mRNA and protein level. Figures 1A and B show that RBM10 was dramatically upregulated in the transfected U2OS cells. Then, a CCK-8 assay was performed to assess the effect of RBM10 on osteosarcoma cell growth. The results showed that U2OS cell growth was significantly inhibited when RBM10 is overexpressed as compared with negative control cells ( Figure 1C, P<0.05). Since anchorage-independent growth is a hallmark of cancer cells [29], we a performed colony formation assay to investigate the role of RBM10 overexpression in anchorage-independent growth of osteosarcoma cells. We found that overexpression of RBM10 almost abrogated U2OS colony formation compared with negative control cells ( Figure 2, P<0.05). Thus, these data suggest that overexpression of RBM10 could suppress the proliferation of human osteosarcoma cells.
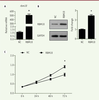 | Figure 1.
Overexpression of RBM10 suppresses growth of U2OS osteosarcoma cells. (A) U2OS cells were transfected with pcDNA3.1-RBM10. RNA was then extracted and the expression of RBM10 was assessed by qPCR. Data are shown as mean ± S.D. of three independent experiments. * P<0.05. (B) RBM10-overexpressed U2OS cells (RBM10) and negative control cells (NC) were lysed and subjected to SDS-PAGE and Western Blotting was performed to detect the expression of RBM10. Data are representative of three independent experiments. * P<0.05. (C) RBM10-overexpressed U2OS cells (RBM10) and negative control cells (NC) were seeded in 96-well plates. CCK-8 assay was performed every day for 4 days. The experiment was performed in quintuplicate. The results are shown as mean ± S.D. * P<0.05. |
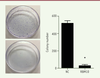 | Figure 2.
Overexpression of RBM10 suppresses colony formation of U2OS osteosarcoma cells. RBM10-overexpressed U2OS cells (RBM10) and negative control cells (NC) were seeded in 6-well plates with soft agar. After incubation for 14 days, a colony formation assay was performed (left) and the number of colonies were calculated (right). Data are shown as mean ± S.D. * P<0.05. |
Overexpression of RBM10 also inhibits the migration and invasion of U2OS osteosarcoma cells. We further evaluated the role of RBM10 overexpression in U2OS cell migration and invasion. As shown in
Figure 3A
, the number of migratory cells significantly (
Figure 3A, right; P<0.05) decreased in RBM10-overexpressed U2OS cells compared with negative control cells ( Figure 3A, left). In invasion experiments, the number of invasive cells was found significantly lower (
Figure 3A right, P<0.05) when RBM10-overexpressed U2OS cells were compared to negative control cells ( Figure 3A, left). These results suggest that overexpression of RBM10 inhibits the migration and invasion ability of human osteosarcoma cells.
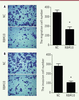 | Figure 3.
Overexpression of RBM10 inhibits the migration and invasion of U2OS osteosarcoma cells. (A) RBM10-overexpressed U2OS cells (RBM10) and negative control cells (NC) were seeded in Transwell chamber overnight. A cell migration assay was then performed (left) and the number of migratory cells was calculated (right). Results are shown as mean ± S.D. * P<0.05. (B) RBM10-overexpressed U2OS cells (RBM10) and negative control cells (NC) were seeded in Matrigel-coated Transwell chamber overnight. Then, a cell invasion assay was performed (left) and the number of migratory cells was assessed (right). The results are shown as mean ± S.D. * P<0.05. |
Overexpression of RBM10 induces U2OS osteosarcoma cell apoptosis and promotes TNF-a production. Cell apoptosis is an important event in cancer progression. To investigate the role of RBM10 in osteosarcoma cell apoptosis, we overexpressed RBM10 in U2OS cells and detect the effect of RBM10 on U2OS cell apoptosis. Overexpression of RBM10 dramatically induced U2OS cell apoptosis compared with negative control cells (
Figure 4A
, P<0.05). We also found that RBM10 overexpression significantly inhibited the expression of Bcl-2 and induced the expression of caspase-3 (
Figure 4B
, P<0.05).
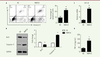 | Figure 4.
Overexpression of RBM10 induces U2OS osteosarcoma cell apoptosis via promoting TNF-a production. (A) U2OS cells were transfected with pcDNA3.1-RBM10. Cells were then harvested, stained with AnnexinV and PI and analyzed by flow cytometry. The results are shown as mean ± S.D. * P<0.05. (B) RBM10-overexpressed U2OS cells (RBM10) and negative control cells (NC) were lysed and subjected to SDS-PAGE and Western Blotting was performed to detect the expression of Bcl-2 and capsase-3. Data are representative of three independent experiments. * P<0.05. (C) RNA was extracted from RBM10-overexpressing U2OS cells (RBM10) and negative control cells (NC). The expression of TNF-α was detected by qPCR. Results are shown as mean ± S.D. * P<0.05. (D) U2OS cells were transfected with pcDNA3.1-RBM10. Supernatants were harvested and TNF-α production was detected by ELISA. The results are shown as mean ± S.D. * P<0.05. |
We also investigated the production of TNF-α by U2OS cells. Overexpression of RBM10 dramatically induced TNF-α production by these cells, both at mRNA and protein level (
Figure 4C and 4D, P<0.05). These data indicated that RBM10 overexpression induced osteosarcoma cell apoptosis via promoting the production of TNF-α that appear to act as an autocrine factor regulating osteosarcoma programmed cell death. |
In this study, we report that RBM10 acts as a tumor suppressor in osteosarcoma via the inhibition of cell growth, cell migration and invasion and the induction of cell apoptosis by inhibiting Bcl-2, activating caspase-3, and producing TNF-α. A number of studies have reported the role of RBM10 in the promotion of cell cycle arrest and apoptosis [12, 24, 25, 30-32]. For example, RBM10 expression correlated with decreased cell proliferation and increased apoptosis in hypertrophic primary chondrocytes [32]. Our results shows that overexpression of RBM10 significantly increases U2OS osteosarcoma cell apoptosis. Apoptosis pathway is divided into intrinsic apoptosis pathway and extrinsic apoptosis pathway [33]. It has been reported that RBM10 mRNA expression correlated with an increased mRNA expression of the pro-apoptotic protein BAX and of the tumor suppressor protein TP53 transcriptional activity in breast cancer [34]. Our results demonstrate that RBM10 overexpression induces osteosarcoma cell apoptosis via the inhibition of Bcl-2 and activation of caspase-3. In addition, a previous study has reported that RBM10 overexpression promotes apoptosis through TNF-α transcription in breast cancer [24]. We show herein that RBM10 overexpression induces osteosarcoma cell apoptosis which is associated with the transcription and production of TNF-α. Although the role of RBM10 in cancer progression is controversial, we elucidate here that RBM10 could act as a tumor suppressor in osteosarcoma cells by inhibiting cell growth, cell migration and invasion and by inducing cell apoptosis. However, we used cells from an osteosarcoma cell line, U2OS, and it is now required to investigate the expression of RBM10 in osteosarcoma, its role in vivo using pre-clinical models and its relationship with tumor stage in patients with osteosarcoma. Our results could provide a novel biomarker for diagnosis and may open new avenues for the treatment of patients with osteosarcoma. |
The authors state that there are no competing interests in this work.
|
1.
Collins
M
Wilhelm
M
Conyers
R
et al. Benefits and adverse events in younger versus older patients receiving neoadjuvant chemotherapy for osteosarcoma: findings from a meta-analysis . Journal of clinical oncology : official journal of the American Society of Clinical Oncology. 2013; ; 31 : :2303.–2312. 2.
Hasei
J
Sasaki
T
Tazawa
H
et al. Dual programmed cell death pathways induced by p53 transactivation overcome resistance to oncolytic adenovirus in human osteosarcoma cells . Molecular cancer therapeutics. 2013; ; 12 : :314.–325. 3.
Mirabello
L
Troisi
RJ
Savage
SA
Osteosarcoma incidence and survival rates from 1973 to 2004: data from the Surveillance, Epidemiology, and End Results Program . Cancer. 2009; ; 115 : :1531.–1543. 4.
Zhou
W
Hao
M
Du
X
et al. Advances in targeted therapy for osteosarcoma . Discovery medicine. 2014; ; 17 : :301.–307. 5.
Sakamoto
A
Iwamoto
Y
Current status and perspectives regarding the treatment of osteo-sarcoma: chemotherapy . Reviews on recent clinical trials. 2008; ; 3 : :228.–231. 6.
Glisovic
T
Bachorik
JL
Yong
J
Dreyfuss
G
RNA-binding proteins and post-transcriptional gene regulation . FEBS letters. 2008; ; 582 : :1977.–1986. 7.
Nagase T, Seki N, Tanaka A, et al. Prediction of the coding sequences of unidentified human genes. IV. The coding sequences of 40 new genes (KIAA0121-KIAA0160) deduced by analysis of cDNA clones from human cell line KG-1 . DNA research : an international journal for rapid publication of reports on genes and genomes. 1995;; 2 : :167.–74, 99–210.
8.
Bechara Elias G, Sebestyén E, Bernardis I, et al. RBM5, 6, and 10 Differentially Regulate NUMB Alternative Splicing to Control Cancer Cell Proliferation . Molecular Cell. 2013;; 52 : :720.–33.
9.
Wang
Y
Gogol-Doring
A
Hu
H
et al. Integrative analysis revealed the molecular mechanism underlying RBM10-mediated splicing regulation . EMBO molecular medicine. 2013; ; 5 : :1431.–1442. 10.
Zheng
S
Damoiseaux
R
Chen
L
Black
DL
A broadly applicable high-throughput screening strategy identifies new regulators of Dlg4 (Psd-95) alternative splicing . Genome research. 2013; ; 23 : :998.–1007. 11.
Inoue
A
Yamamoto
N
Kimura
M
et al. RBM10 regulates alternative splicing . FEBS letters. 2014; ; 588 : :942.–947. 12.
Hernandez
J
Bechara
E
Schlesinger
D
et al. Tumor suppressor properties of the splicing regulatory factor RBM10 . RNA biology. 2016; ; 13 : :466.–472. 13.
Atsumi
T
Suzuki
H
Jiang
JJ
et al. Rbm10 regulates inflammation development via alternative splicing of Dnmt3b . International immunology. 2017; ; 29 : :581.–591. 14.
Sutherland
LC
Thibault
P
Durand
M
et al. Splicing arrays reveal novel RBM10 targets, including SMN2 pre-mRNA . BMC molecular biology. 2017; ; 18 : :19.. 15.
Vinayanuwattikun
C
Le Calvez-Kelm
F
Abedi-Ardekani
B
et al. Elucidating Genomic Characteristics of Lung Cancer Progression from In Situ to Invasive Adenocarcinoma . Scientific reports. 2016; ; 6 : :31628.. 16.
Imielinski
M
Berger
AH
Hammerman
PS
et al. Mapping the hallmarks of lung adenocarcinoma with massively parallel sequencing . Cell. 2012; ; 150 : :1107.–1120. 17.
Sebestyen
E
Singh
B
Minana
B
et al. Large-scale analysis of genome and transcriptome alterations in multiple tumors unveils novel cancer-relevant splicing networks . Genome research. 2016; ; 26 : :732.–744. 18.
Giannakis
M
Mu
XJ
Shukla
SA
et al. Genomic Correlates of Immune-Cell Infiltrates in Colorectal Carcinoma . Cell reports. 2016; ; 15 : :857.–865. 19.
Furukawa
T
Kuboki
Y
Tanji
E
et al. Whole-exome sequencing uncovers frequent GNAS mutations in intraductal papillary mucinous neoplasms of the pancreas . Scientific reports. 2011; ; 1 : :161.. 20.
Witkiewicz
AK
McMillan
EA
Balaji
U
et al. Whole-exome sequencing of pancreatic cancer defines genetic diversity and therapeutic targets . Nature communications. 2015; ; 6 : :6744.. 21.
Ibrahimpasic
T
Xu
B
Landa
I
et al. Genomic Alterations in Fatal Forms of Non-Anaplastic Thyroid Cancer: Identification of MED12 and RBM10 as Novel Thyroid Cancer Genes Associated with Tumor Virulence . Clinical cancer research : an official journal of the American Association for Cancer Research. 2017; ; 23 : :5970.–5980. 22.
Lukong
KE
Chang
KW
Khandjian
EW
Richard
S
RNA-binding proteins in human genetic disease . Trends in genetics : TIG. 2008; ; 24 : :416.–425. 23.
Guan
G
Li
R
Tang
W
et al. Expression of RNA-binding motif 10 is associated with advanced tumor stage and malignant behaviors of lung adenocarcinoma cancer cells . Tumour biology : the journal of the International Society for Oncodevelopmental Biology and Medicine. 2017; ; 39 : :1010428317691740.. 24.
Wang
K
Bacon
ML
Tessier
JJ
et al. RBM10 Modulates Apoptosis and Influences TNF-alpha Gene Expression . Journal of cell death. 2012; ; 5 : :1.–19. 25.
Zhao
J
Sun
Y
Huang
Y
et al. Functional analysis reveals that RBM10 mutations contribute to lung adenocarcinoma pathogenesis by deregulating splicing . Scientific reports. 2017; ; 7 : :40488.. 26.
Loiselle
JJ
Roy
JG
Sutherland
LC
RBM10 promotes transformation-associated processes in small cell lung cancer and is directly regulated by RBM5 . PloS one. 2017; ; 12 : :e0180258.. 27.
Steeg
PS
Tumor metastasis: mechanistic insights and clinical challenges . Nature medicine. 2006; ; 12 : :895.–904. 28.
Chambers
AF
Groom
AC
MacDonald
IC
Dissemination and growth of cancer cells in metastatic sites . Nature reviews Cancer. 2002; ; 2 : :563.–572. 29.
Rotem
A
Janzer
A
Izar
B
et al. Alternative to the soft-agar assay that permits high-throughput drug and genetic screens for cellular transformation . Proceedings of the National Academy of Sciences of the United States of America. 2015; ; 112 : :5708.–5713. 30.
Ji
Y
Xie
S
Jiang
L
et al. Increased cell apoptosis in human lung adenocarcinoma and in vivo tumor growth inhibition by RBM10, a tumor suppressor gene . Oncology letters. 2017; ; 14 : :4663.–4669. 31.
Bechara
EG
Sebestyen
E
Bernardis
I
et al. RBM5, 6, and 10 differentially regulate NUMB alternative splicing to control cancer cell proliferation . Mol Cell. 2013; ; 52 : :720.–733. 32.
James
CG
Ulici
V
Tuckermann
J
et al. Expression profiling of Dexamethasone-treated primary chondrocytes identifies targets of glucocorticoid signalling in endochondral bone development . BMC genomics. 2007; ; 8 : :205.. 33.
Kiraz
Y
Adan
A
Kartal Yandim M, Baran Y. Major apoptotic mechanisms and genes involved in apoptosis . Tumour biology : the journal of the International Society for Oncodevelopmental Biology and Medicine. 2016; ; 37 : :8471.–8486. 34.
Martinez-Arribas
F
Agudo
D
Pollan
M
et al. Positive correlation between the expression of X-chromosome RBM genes (RBMX, RBM3, RBM10) and the proapoptotic Bax gene in human breast cancer . Journal of cellular biochemistry. 2006; ; 97 : :1275.–1282. |